2019 Talk Abstracts
Gentle measurement of quantum states and differential privacy
Presenting Author: Scott Aaronson, University of Texas, Austin
In differential privacy (DP), we want to query a database about n users, in a way that "leaks at most ϵabout any individual user," conditioned on any outcome of the query. Meanwhile, in gentle measurement, we want to measure n quantum states, in a way that "damages the states by at most α," conditioned on any outcome of the measurement. In both cases, we can achieve the goal by techniques like deliberately adding noise to the outcome before returning it. We prove a new and general connection between the two subjects. Specifically, on products of n quantum states, any measurement that is α-gentle for small α is also O(α)-DP, and any product measurement that is ϵ-DP is also O(ϵ√n) -gentle.
Illustrating the power of this connection, we apply it to the recently studied problem of shadow tomography. Given an unknown d-dimensional quantum state ρ, as well as known two-outcome measurements E1,...,Em, shadow tomography asks us to estimate Pr[E1 accepts ρ], for every i ∈[m], by measuring few copies of ρ. Using our connection theorem, together with a quantum analog of the so-called private multiplicative weights algorithm of Hardt and Rothblum, we give a protocol to solve this problem using O((logm)2(logd)2) copies of ρ, compared to Aaronson's previous bound of Õ((logm)4(logd)). Our protocol has the advantages of being online (that is, the E1's are processed one at a time), gentle, and conceptually simple.
Characterizing and developing bosonic error-correcting codes
Presenting Author: Victor V. Albert, California Institute of Technology
Continuous-variable or bosonic quantum information processing is a field concerned with using one or more harmonic oscillators to protect, manipulate, and transport quantum information. The large oscillator Hilbert space provides alternative encodings that are currently outperforming encodings into registers of many qubits: break-even error correction has been achieved with the bosonic cat codes but not yet with a many-qubit system. However, an analysis of theoretical capabilities of bosonic codes is missing. We have undertaken a program identifying
- Which codes are able to protect against dominant noise in realistic bosonic systems?
- Why those codes perform so well?
- How to extend codes to multiple modes advantageously?
We provide answers to all these questions. First, we calculate the error-correction conditions of single-mode codes, showing that Gottesman-Kitaev-Preskill (GKP) codes offer the best performance. Second, we prove that GKP codes achieve the quantum capacity (up to a constant offset) of the thermal loss channel. Third, we present a multimode extension of the cat codes that increases both experimental feasibility and theoretically achievable performance.
Things you can do with your quantum key distribution setup: signatures and oblivious transfer
Presenting Author: Erika Andersson, Herriot-Watt University
Modern cryptography is more than encryption, and quantum cryptography is more than quantum key distribution. "Quantum digital signatures” were first proposed by Gottesman and Chuang in 2001, inspired by public-key signature schemes. Broadly speaking, a signature guarantees that a message cannot be altered or forged. There can be more than one possible recipient, and messages can be forwarded from one recipient to another. The first quantum signature scheme developed into something more practical, essentially using the same experimental components as quantum key distribution. This led to realisations of measurement-device-independent quantum signatures at Toshiba, Cambridge, and by J-W Pan’s group in China. Oblivious transfer is another functionality different from encryption. In 1-out-of-2 oblivious transfer, a receiver obtains only one of two bits sent by a sender. The sender does not know which of the two bits the receiver obtains, and the receiver does not know the other bit. Such a “poor communication channel” is, perhaps surprisingly, an important primitive for secure multiparty computation. Quantum oblivious transfer is possible, but with some limitations. In the second half of this talk, I will describe a scheme for quantum oblivious transfer that works at least as well than any of the previous ones, and which only needs the same components as standard quantum key distribution.
Quantum optical fingerprinting without a shared phase reference
Presenting Author: Konrad Banaszek, University of Warsaw
Quantum fingerprinting allows two remote parties to determine whether their datasets are identical or different by transmitting exponentially less information compared to the classical protocol with equivalent performance. Standard optical implementations of quantum fingerprinting based on coherent states of light require phase stability between the sending parties. Here we present a quantum fingerprinting protocol which exploits higher-order optical interference between optical signals with a random global phase. Its performance has been verified in a proof-of-principle experiment discriminating between binary visibility hypotheses. Actual demonstration of quantum advantage over the known bound on the performance of classical fingerprinting protocols should be possible using currently available technology.
Bang-bang control as a design principle for classical and quantum optimization algorithms
Presenting Author: Aniruddha Bapat, University of Maryland Joint Quantum Institute
Physically motivated classical heuristic optimization algorithms such as simulated annealing (SA) treat the objective function as an energy landscape, and allow walkers to escape local minima. It has been speculated that quantum properties such as tunneling may give quantum algorithms the upper hand in finding ground states of vast, rugged cost landscapes. Indeed, the Quantum Adiabatic Algorithm (QAO) and the recent Quantum Approximate Optimization Algorithm (QAOA) have shown promising results on various problem instance that are considered classically hard. Here, we argue that the type of \emph{control} strategy used by the optimization algorithm may be crucial to its success, both classically and quantumly. Along with SA, QAO and QAOA, we define a new, bang-bang version of simulated annealing, BBSA, and study the performance of these algorithms on two well-studied problem instances from the literature. Rather than a quantum advantage, we find evidence for a design advantage. Both classically and quantumly, the successful control strategy is found to be bang-bang, exponentially outperforming the annealing analogues on the same instances. Lastly, we construct O(1) time QAOA protocols for a large class of symmetric cost functions, and provide an accompanying physical picture.
Quantum control and simulation with large trapped-ion crystals
Presenting Author: John Bollinger, National Institute of Standards and Technology, Boulde
I will describe efforts to improve the control of large, single-plane crystals of several hundred ions in Penning traps and employ these crystals for quantum sensing and quantum simulation. We isolate and control two internal levels or “spin” degree of freedom in each ion with standard techniques. Long-range interactions between the ions are generated through the application of spin-dependent optical-dipole forces that couple the spin and motional degrees of freedom of the ions. When this coupling is tuned to produce a coupling with a single motional mode (typically the center-of-mass mode), this system is described by the iconic Dicke model. Long-range Ising interactions and single-axis twisting are produced through this spin-motion coupling. To benchmark dynamics, we measure out-of-time-order correlations (OTOCs) that quantify the build-up of correlations and the spread of quantum information. We also employ spin-dependent optical dipole forces to sense center-of-mass motion that is small compared to the ground state zero-point fluctuations. This enables the detection of weak electric fields and may provide an opportunity to place limits on dark matter couplings due to particles such as axions and hidden photons that couple to ordinary matter through weak electric fields.
Randomized benchmarking under different gatesets
Presenting Author: Kristine Boone, University of Waterloo
We provide a comprehensive analysis of the differences between two important standards for randomized benchmarking (RB): the Clifford-group RB protocol proposed originally in Emerson et al (2005) and Dankert et al (2006), and a variant of that RB protocol proposed later by the NIST group in Knill et al, PRA (2008). While these two protocols are frequently conflated or presumed equivalent, we prove that they produce distinct exponential fidelity decays leading to differences of up to a factor of 3 in the estimated error rates under experimentally realistic conditions. These differences arise because the NIST RB protocol does not satisfy the unitary two-design condition for the twirl in the Clifford-group protocol and thus the decay rate depends on non-invariant features of the error model. Our analysis provides an important first step towards developing definitive standards for benchmarking quantum gates and a more rigorous theoretical underpinning for the NIST protocol and other RB protocols lacking a group-structure. We conclude by discussing the potential impact of these differences for estimating fault-tolerant overheads.
Uncloneable encryption
Presenting Author: Anne Broadbent, University of Ottawa
In 2002, Gottesman answered this question in the positive, proposing a quantum encryption scheme for classical messages, with a decryption process that detects any attempt to copy the ciphertext. Clearly, classical information alone does not allow such a functionality, since it is always possible to perfectly copy a classical ciphertext while avoiding detection. However, Gottesman left open the question of restricting the knowledge that two recipients could simultaneously have on a plaintext, after an attack on a single ciphertext. Here, we address this open question by showing that Wiesner's conjugate coding can be used to achieve this type of uncloneable encryption for classical messages. Our approach is a prepare-and-measure scheme and the analysis is done in the quantum random oracle model, using techniques from the analysis of monogamy-of-entanglement games.
Engineering atom-light interactions in photonic crystal waveguides
Presenting Author: Alex Burgers, California Institute of Technology
Integrating cold atoms with nanophotonics enables the exploration of new paradigms in quantum optics and many body physics. Advanced fabrication capabilities for low-loss dielectric materials provide powerful tools to engineer band structure and light-matter couplings between photons and atoms. The current system at Caltech to explore such phenomena consists of a quasi-one-dimensional photonic crystal waveguide (PCW) whose band structure arises from periodic modulation of the dielectric structure. The waveguide design gives rise to stable trap sites for atoms at each unit cell of the crystal (150 sites for the 1D waveguide). Atoms localized in these traps will interact with one another via guided modes of the waveguide creating a versatile system that can be utilized for both quantum memories and quantum simulation. We have performed extensive trajectory simulations of atoms delivered by an optical lattice to the PCWs. The good correspondence between simulation and data enables us to understand the microscopic dynamics of atoms near the waveguide and introduce auxiliary GMs that perturb the atoms and reveal how they can be delivered to these GM trap regions. I will present recent efforts to achieve high fractional filling of trap sites within the PCW using the optical lattice delivery system and discuss future research goals.
Monitored quantum jumps: The view from quantum trajectory theory
Presenting Author: Howard Carmichael, University of Auckland
Quantum jumps are emblematic of all things quantum. Certainly that is so in the popular mind…and more than just an echo from the past, the term “quantum jump” still holds a prominent position within the lexicon of modern physics. What, however, is the character of the jump on close inspection? Is it discontinuous and discrete, as in Bohr’s original conception? Or is it some form of continuous Schrödinger evolution that might be monitored and reconstructed, even interrupted and turned around? I consider the jumps of single trapped ions observed in the mid-1980s [1], where an understanding drawn from quantum trajectory theory favours the latter option. I present that understanding and its connection to the modern view of continuous quantum measurement, and support this view from the theory side with experimental results [2], which recover the continuous and deterministic path of quantum jumps in a superconducting circuit using conditional quantum state tomography. [1] W. Nagourney et al., Phys. Rev. Lett. 56, 2797 (1986); T. Sauter et al., Phys. Rev. Lett. 57, 1696 (1986); J. C. Bergquist et al., Phys. Rev. Lett. 57, 1699 (1986). [2] Z. K. Minev, S. O. Mundhada, S. Shankar, P. Rheinhold, R. Gutiérrez-Jáuregui, R. J. Schoelkopf, M. Mirrahimi, H. J. Carmichael, and M. H. Devoret, arXiv:1803.00545 (2018).
Time-bin and polarization superdense teleportation for space applications
Presenting Author: Joseph Chapman, University of Illinois at Urbana-Champaign
To build a global quantum communication network, low-transmission, fiber-based communication channels can be supplemented by using a free-space channel between a satellite and a ground station on Earth. We have constructed a system that generates hyperentangled photonic "ququarts'' and measures them to execute multiple quantum communication protocols of interest. We have successfully executed and characterized superdense teleportation---our measurements show an average fidelity of 0.94±0.02, with a phase resolution under 7° allowing reliable transmission of >10^5 distinguishable quantum states. Additionally, we have demonstrated the ability to compensate for the Doppler shift, which would otherwise prevent sending time-bin encoded states from a rapidly moving satellite, thus allowing the low-error execution of phase-sensitive protocols during an orbital pass.
Learning short- and constant-depth algorithms: application to state overlap and entanglement spectroscopy
Presenting Author: Lukasz Cincio, Los Alamos National Laboratory
Short-depth algorithms are crucial for reducing computational error on near-term quantum computers, for which decoherence and gate infidelity remain important issues. Here we present a machine-learning approach for discovering such algorithms. We apply our method to a ubiquitous primitive: computing the overlap ${\rm Tr}(\rho\sigma)$ between two quantum states $\rho$ and $\sigma$. The standard algorithm for this task, known as the Swap Test, is used in many applications such as quantum support vector machines. Here, our machine-learning approach finds algorithms that have shorter depths than the Swap Test, including one that has a constant depth (independent of problem size). Taking this as inspiration, we also present a novel constant-depth algorithm for computing the integer R\'enyi entropies, ${\rm Tr}(\rho^n})$, where our circuit depth is independent of both the number of qubits in $\rho$ as well as the exponent $n$. These integer R\'enyi entropies are useful, e.g., for computing the entanglement spectrum for condensed matter applications. Finally, we demonstrate that both our state overlap algorithm and our R\'enyi entropy algorithm have increased robustness to noise relative to their state-of-the-art counterparts in the literature.
Simulating and evaluating the coherent Ising machine
Presenting Author: Noah Davis, University of Texas, Austin
Physical annealing techniques present methods for taking advantage of qubits without the need for universal quantum computers. Particularly, annealing systems may offer calculation speed-ups for certain NP-hard optimization problems such as the Max-Cut problem and the Sherrington-Kirkpatrick model. Among promising annealing systems, the coherent Ising machine (CIM) has demonstrated particular potential for solving dense examples of these problems. A CIM uses classical measurement and feedback to couple the degenerate optical parametric oscillators which make up its logical qubits. We use the master equations governing this measurement-feedback system to simulate an idealized (but still classically controlled) CIM on a high performance computing cluster. We present an analysis of this simulation and compare it to experimental instances of CIMs along with other popular annealing methods.
Quantum simulation with alkali and alkaline-earth Rydberg-arrays
Presenting Author: Manuel Endres, California Institute of Technology
Recently, cold alkali atoms in optical tweezer arrays have emerged as a versatile platform for quantum simulation. I will review these developments and give an update about ongoing experiments with alkaline-earth atoms:
- I will introduce atom-by-atom assembly as a fast and simple method to generate defect-free atomic arrays.
- I will review how such arrays can be used as a quantum simulator for specific types of transverse- & longitudinal-field Ising-models with 1/R^6 interaction.
- I will outline how we are currently extending this work to alkaline-earth atoms using Strontium-88; particularly, I will illustrate how this new direction could overcome current coherence limits and enable scalability to larger tweezer arrays.
Operational, gauge-free quantum tomography
Presenting Author: John Gamble, Microsoft Research
As quantum processors become increasingly refined, benchmarking them in useful ways becomes a critical topic. Traditional approaches to quantum tomography, such as state tomography, suffer from self-consistency problems, requiring either perfectly pre-calibrated operations or measurements. This problem has recently been tackled by explicitly self-consistent protocols such as randomized benchmarking, robust phase estimation, and gate set tomography (GST). An undesired side-effect of self-consistency is the presence of gauge degrees of freedom, arising from the lack fiducial reference frames, and leading to large families of gauge-equivalent descriptions of a quantum gate set which are difficult to interpret. We solve this problem through introducing a gauge-free representation of a quantum gate set inspired by linear inversion GST. This allows for the efficient computation of any experimental frequency without a gauge fixing procedure. We use this approach to implement a Bayesian version of GST using the particle filter approach, which was previously not possible due to the gauge. Within Bayesian GST, the prior information allows for inference on tomographically incomplete data sets, such as Ramsey experiments, without giving up self-consistency. We demonstrate simulated examples of this approach for a variety of experimentally-relevant situations, showing the stability and generality of both our gauge-free representation and Bayesian GST.
Chaos, stability and quantum-classical correspondence in spin systems
Presenting Author: Shohini Ghose, Wilfrid Laurier University
Classical chaos is characterized by extreme sensitivity of a system's dynamics to small perturbations in initial conditions. At the quantum level, a similar characterization of chaos remains a challenge due to the uncertainty principle and the apparent linearity of quantum evolution. We have explored the question of quantum chaos in spin systems both theory and experiments. Various signatures of chaos and classical bifurcations can be observed in a deeply quantum regime as well as the semiclassical regime. Chaos can affect quantum phenomena such as entanglement and fidelity decay that play important roles in quantum information processing. We present a method to quantify the Bohr correspondence principle in chaotic systems, and explain previous conflicting results regarding the connection between chaos and entanglement.
Photonic quantum computing
Presenting Author: Mercedes Gimeno-Segovia, PsiQuantum Corp.
Photons make great qubits, they are cheap to produce, resilient to noise and the only known option for quantum communication. The two main traditional arguments against a fully linear-optical quantum computing architecture have been the lack of deterministic photonic entangling gates and the predisposition of photons to loss. However, a number of theoretical breakthroughs have made these arguments loose strength, while implementations in silicon photonics have opened the door to manufacturability at large scale. In this talk, I will describe an architecture for fault tolerant quantum computing based on linear optics, in the process I will explain how measurement-induced non-linearity can overcome the challenge of creating entanglement and how loss can be tackled with well-known error correcting codes.
Noisy circuit training of generative models with superconducting qubits
Presenting Author: Kathleen Hamilton, Oak Ridge National Laboratory
Many NISQ devices with < 20 qubits are becoming available for public use, but the lack of detailed noise models makes it difficult to use simulation to predict performance on hardware. We have used a recently introduced class of generative models [1] to quantify the performance of noisy superconducting qubits. Many sources of error and noise can be identified (e.g. decoherence, gate fidelities and measurement errors), and while the use of noise-robust stochastic optimizers can train circuits to a reasonable degree of accuracy, the cohesive incorporation of error mitigation into circuit training remains an open question. Our work focuses on how the performance of generative models on noisy qubits can be improved without error mitigation: by minimizing the number of noisy gates in a circuit and using sampling rates to improve the dynamics of gradient-based circuit training. [1] Liu, Jin-Guo, and Lei Wang. "Differentiable learning of quantum circuit Born machine." arXiv:1804.04168 (2018). This work was supported as part of the ASCR Testbed Pathfinder Program at Oak Ridge National Laboratory under FWP #ERKJ332
Quantum error correction in quantum gravity
Presenting Author: Patrick Hayden, Stanford University
TBA
New frontiers in laser cooling of neutral atoms and trapped ions
Presenting Author: Murray Holland, University of Colorado JILA
We theoretically analyze the novel physics in two recent demonstrations of laser cooling [1,2]. First, we describe laser cooling by Sawtooth Wave Adiabatic Passage (SWAP) of neutral atoms in free space that possess narrow linewidth transitions [3]. SWAP cooling exploits the extreme coherence on offer using near-resonant laser fields whose frequencies are time dependent. With a reduced reliance on spontaneous emission compared to Doppler cooling, SWAP cooling shows promise for cooling systems lacking closed cycling transitions, such as molecules. Second, we numerically investigate the efficiency of near ground-state cooling of large 2D ion crystals in Penning traps using electromagnetically induced transparency (EIT) [4]. We show that, in spite of the challenges posed by these rotating multi-ion crystals, the large bandwidth of drumhead modes (hundreds of kilohertz) can be rapidly cooled to near ground-state occupations. We predict a surprising enhancement of the cooling rate of the center-of-mass mode with increasing number of ions. We will highlight relevant experimental results in support of our theories. [1] M. Norcia et al. New J. Phys. 20 (2018) [2] E. Jordan et al. arXiv:1809.06346 (Sep. 2018) [3] J.P. Bartolotta et al. Phys. Rev. A 98 (2018) [4] A. Shankar et al. arXiv:1809.05492 (2018)
Novel trap for 2D ion crystal experiments
Presenting Author: Megan Ivory, University of Washington
Quantum computation has thus far been limited by number of available qubits. In trapped ions, most computation has been performed in linear Paul traps to avoid micromotion which is thought to lead to low gate fidelities. Recent theoretical work by the Duan group shows that micromotion can be compensated with the use of segmented laser pulses, allowing for fidelities <99.99% in two-dimensional ion crystals of >100 ions. Here, we seek to experimentally demonstrate high-fidelity quantum gates in Ba+ ions in a planar crystal. To do so, we have developed a novel trap system specifically for producing Ba+ crystals. The trap geometry is based on simulations we developed for modeling trapped ion dynamics and equilibrium positions. The electrodes are comprised of a segmented ring which allows us to dynamically tune the transverse trap frequencies to produce both planar and linear traps. We present progress towards the demonstration of large ion crystals of varying trap frequency anisotropies. In addition to high-fidelity quantum gates in planar ion crystals, the system in development can also be used for quantum chemistry simulations and the study of crystalline order, defects, and phase transitions.
Quantum simulation of fermions: geometric locality and error mitigation
Presenting Author: Zhang Jiang, Google
We consider mappings from fermionic systems to spin systems that preserve geometric locality in more than one spatial dimension. They are useful to simulating lattice fermionic systems on a quantum computer, e.g., the Hubbard model. Locality-preserving mappings avoid the large overhead associated with the nonlocal parity terms in conventional mappings, such as the Jordan-Wigner transformation. As a result, they often provide solutions with much lower circuit depths. Here, we construct locality-preserving mappings that can also detect/correct single-qubit errors without introducing extra physical qubits beyond those required by the original mappings. We discuss error mitigation strategies based on these encodings for quantum algorithms such as the variational quantum eigensolver.
Spin-blockade spectroscopy of Si/SiGe quantum dots
Presenting Author: Tyler Keating, HRL Laboratories
Many exchange-based platforms for spin qubits rely on spin-to-charge conversion for initialization and readout. The robustness of spin-to-charge conversion depends on the singlet-triplet energy splitting of two electrons occupying one dot, which is set by the energy of the dot's lowest-lying excited state. We demonstrate a model-independent technique to measure this energy, using repeated single-shot measurement across the spin-to-charge window. In our Si/SiGe triple dot device, we find that excitation energies vary smoothly with nearby gate bias, suggesting that the lowest-lying states are orbital in character. We also consider other, model-specific parameters that could be extracted from this type of measurement.
Stationary phase method in discrete Wigner functions and classical simulation of quantum circuits
Presenting Author: Lucas Kocia, National Institute of Standards and Technology, Maryland
We apply the periodized stationary phase method to discrete Wigner functions of systems with odd prime dimension using results from -adic number theory. We derive the Wigner-Weyl-Moyal (WWM) formalism with higher order corrections representing contextual corrections to non-contextual Clifford operations. We apply this formalism to a subset of unitaries that include diagonal gates such as the gates. We characterize the stationary phase critical points as a quantum resource injecting contextuality and show that this resource allows for the replacement of the points that represent magic state Wigner functions on -dimensional qudits by points. We find that the gate introduces the smallest higher order correction possible, requiring the lowest number of additional critical points compared to the Clifford gates. We then establish a relationship between the stabilizer rank of states and the number of critical points and exploit the stabilizer rank decomposition of two qutrit gates to develop a classical strong simulation of a single qutrit marginal on qutrit gates that are followed by Clifford evolution, and show that this only requires calculating critical points corresponding to Gauss sums. This outperforms the best alternative qutrit algorithm for any number of
Dephrasure channel and superadditivity of coherent information
Presenting Author: Felix Leditzky, University of Colorado JILA
The quantum capacity of a quantum channel captures its capability for noiseless quantum communication. It lies at the heart of quantum information theory. Unfortunately, our poor understanding of nonadditivity of coherent information makes it hard to understand the quantum capacity of all but very special channels. In this paper, we consider the dephrasure channel, which is the concatenation of a dephasing channel and an erasure channel. This very simple channel displays remarkably rich and exotic properties: we find nonadditivity of coherent information at the two-letter level, a big gap between single-letter coherent and private informations, and positive quantum capacity for all complementary channels. Its clean form simplifies the evaluation of coherent information substantially and, as such, we hope that the dephrasure channel will provide a much-needed laboratory for the testing of new ideas about nonadditivity.
What a small-scale, highly-accurate quantum processor can teach us about analog quantum simulation
Presenting Author: Nathan Lysne, University of Arizona
Quantum systems that offer reasonably accurate control over tens of qubits have now been realized in several contexts. It is thought such noisy intermediate-scale quantum (NISQ) devices may be capable of classically hard tasks such as analog quantum simulation (AQS). Yet, it remains unclear if a quantum processor without error correction and fault tolerance can compute meaningful results when subject to realistic imperfections. To probe this question we have developed a universal, highly accurate analog quantum processor operating in the 16D Hilbert space comprised of the total atomic spin of individual Cs atoms in the electronic ground state. Advances in optimal control enables us to drive arbitrary unitary transformations with very high fidelity (>99%) which we can use to perform simulations of any quantum system that fits in this Hilbert space. In particular, we have studied the feasibility of simulating several model Hamiltonians that exhibit features of interest to AQS, such as chaos and hypersensitivity (the quantum kicked top), and quantum phase transitions (the Lipkin-Meshkov-Glick and transverse Ising models). Experimentally, we demonstrate AQS of each of these models, with high fidelity at the quantum state level and accurate tracking of dynamical features. With this small-scale highly accurate quantum simulator, we can now reintroduce errors in a controlled fashion and study how they impact AQS of complex dynamics, in the laboratory as well as numerical modeling.
Sensing near the Heisenberg limit with a trapped-ion mechanical oscillator
Presenting Author: Katherine McCormick, University of Colorado
Developing tools for precisely controlling and measuring the motion of a trapped ion could contribute to several possible applications, such as improving fidelities of quantum computations, opening up new avenues for quantum simulations and using ions as quantum-mechanical sensors in searches for new physics. I will discuss recent work aimed at characterizing and improving the level of motional coherence in trapped-ion systems, and present potential extensions for precision measurement applications. First, I will present results on the generation of oscillator number states up to n = 100 and superpositions of the form |0⟩+|n⟩, with n up to 18. These superposition states are used to measure the motional frequency with a sensitivity that ideally follows the 1/n Heisenberg scaling. Second, we investigate the spectrum of motional frequency noise using a series of coherent displacements of the motion of the ion, with features similar to Ramsey and dynamical decoupling sequences. These techniques, while demonstrated in a trapped-ion system, should be widely adaptable to other quantum-mechanical harmonic oscillators.
Improving the sensitivity of Advanced LIGO with squeezed light
Presenting Author: Lee McCuller, Massachusetts Institute of Technology
The upcoming observing run 3 of Advanced LIGO will include quantum squeezed light at both observatories to improve sensitivity. This talk will detail the ongoing commissioning, as well as the technical requirements driving the design and control of audio-band squeezed light sources for gravitational wave optical interferometers. In addition, R&D is ongoing to demonstrate frequency dependent squeezed vacuum at 50Hz using optical filter cavities. Such an implementation will allow LIGO to reduce quantum radiation pressure noise below the standard quantum limit to achieve improvements spanning its observation band.
Entanglement properties of quantum random walks
Presenting Author: Alexander Meill, University of California San Diego
We examine the entanglement assumptions used to derive dynamics in highly symmetric quantum random walks.
Entangled-state measurements based on mode-resolved two-photon sum-frequency generation
Presenting Author: Sofiane Merkouche, University of Oregon
Projective measurements onto entangled quantum states (commonly referred to as "entangled measurements") are an essential tool for many quantum information processing applications, for example quantum repeaters and quantum-state teleportation. The most well-studied such measurement is the Bell-state measurement for two qubits. Here, we introduce a two-photon multi-mode entangled-state measurement scheme based upon sum-frequency generation (SFG) followed by mode-resolved single-photon detection. We show that the mode-resolved detection of the output of a two-photon SFG process acts as a projective measurement onto the two-photon entangled state produced by the time-reversed parametric downconversion process in the perturbative limit. We analyze the applicability of such a measurement both for temporal- and spatial-mode entanglement, and show how this can be exploited for high-dimensional quantum teleportation, entanglement swapping, and quantum illumination.
Quantum computational supremacy in the sampling of Bosonic random walkers on a one-dimensional lattice
Presenting Author: Gopikrishnan Muraleedharan, University of New Mexico CQuIC
A quantum device that performa a computational task more efficiently than a current state-of-the-art classical computer is said to demonstrate quantum computational supremacy QCS. One path to achieving QCS in the short term is via sampling complexity; random samples are drawn from a probability distribution by measuring a complex quantum state in a defined basis. Surprisingly, a gas of identical noninteracting bosons can yield sampling complexity due solely to quantum statistics, as shown by Aaronson and Arkhipov, and dubbed boson sampling the context of identical photons scattering from a linear optical network. We generalize this to noninteracting bosonic quantum random walkers on a 1D lattice, and study the complexity of the resulting probability distribution obtained in static and time dependent lattices. We consider physical realizations based on controlled transport of ultra-cold atoms in a spinor optical lattice as well as a quantum gas microscope using optical tweezers. We quantify analytically and numerically how a sequence of random Hamiltonian evolution approaches Haar random SU (\(d\)) unitary. This, together with identical particle interference can yield QCS. We also study how much pseudorandomness is necessary to demonstrate QCS in terms of closeness to a t-design.
Electric-field noise from thermally-activated fluctuators in a surface ion trap
Presenting Author: Crystal Noel, University of California Berkeley
Electric field noise is a major limiting factor in the performance of ion traps and other quantum devices. Despite intensive research over the past decade, the nature and cause of electric field noise near surfaces is not very well understood. We probe electric-field noise near the surface of an ion trap chip in a previously unexplored high-temperature regime. A saturation of the noise amplitude occurs around 500 K, which, together with a small change in the frequency scaling, points to thermally activated fluctuators as the origin of the noise. The data can be explained by a broad distribution of activation energies around 0.5 eV. These energies suggest atomic displacements as a relevant microscopic mechanism, likely taking place at the metal surface.
Unusual entanglement dynamics in the quantum kicked top
Presenting Author: Arjendu Pattanayak, Carleton College
We study the quantum kicked top in the experimentally accessible regime of a few qubits \(N \in \{2, 8\}\). We focus on the entanglement dynamics \(|\psi(t)>\) of intial spin coherent states on the \((J_x,J_y,J_z)\) sphere. We demonstrate that the quantum behavior at a given location can correlate with, or anti-correlate with, or be decorrelated with the limiting \(N \to \infty\) classical phase-space behavior. Globally, quantum spectra and eigenfunctions visualized via expansion coefficients in the Hilbert space of the \(J_z\) operator are shown to be periodic in \(K\) whence the quantum dynamics are (quasi-)periodic in time \(T\) and nonlinear kick strength \(K\), unlike the classical dynamics although decoherence distinguishes between different \(K\) regimes. Further, there are patterns in the quantum dynamics that repeat as a function of \(N\). We explore novel oscillations where |\(\psi>\) moves between two maximally entangled (GHZ-like) configurations \((|\chi_+>, |\chi_->)\) which occur for \(N=4,8\) in our system. We show that linear combinations of the \(\chi\) states relax to different final entangled states for a decoherent Kraus map of weighted sum of Floquet operators. Thus quantum entanglement for a classically chaotic system can depend on initial conditions (but not as for the classical system) and can yield final high entanglement even for states 'thermalized' under decoherence. We connect to the classical phase-space dynamics via the Husimi projections of these \(\chi\) states.
Towards microwave assisted spin-spin entanglement
Presenting Author: Jason Petta, Princeton University ()
Electron spins are excellent candidates for solid state quantum computing due to their exceptionally long quantum coherence times, which is a result of weak coupling to environmental degrees of freedom. However, this isolation comes with a cost, as it is difficult to coherently couple two spins in the solid state, especially when they are separated by a large distance. Here we combine a large electric-dipole interaction with spin-orbit coupling to achieve spin-photon coupling. Vacuum Rabi splitting is observed in the cavity transmission as the Zeeman splitting of a single spin is tuned into resonance with the cavity photon. We achieve a spin-photon coupling rate as large as \(g _s/2 {\pi}\) = 10 MHz, which exceeds both the cavity decay rate \({\kappa}/2{\pi}\) = 1.8 MHz and spin dephasing rate \({\gamma}/2{\pi}\) = 2.4 MHz, firmly anchoring our system in the strong-coupling regime. Moreover, the spin-photon coupling mechanism can be turned off by localizing the spin in one side of the double quantum dot. Recent progress towards microwave assisted spin-spin entanglement will be presented.
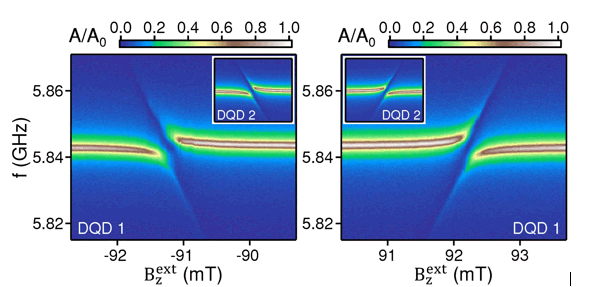
Feedback-based simulation of quantum nonlinear dynamics: The Quantum Kicked Top in an ensemble of two-level atoms
Presenting Author: Pablo Poggi, University of New Mexico CQuIC
We study the implementation of a measurement-based feedback scheme to realize quantum nonlinear dynamics. We specifically study the Quantum Kicked Top (QKT), a standard paradigm of quantum chaos, in the context of an ensemble of spins. The scheme uses a sequence of (not-so) weak measurements of a collective spin variable and global rotations conditioned on the measurement outcome. We show that the resulting dynamics, ensemble averaged over many realizations, is governed by a combination of the QKT Hamiltonian and a non-unitary channel which vanishes in the semiclassical limit, recovering the Classical Kicked Top. We also analyze individual quantum trajectories, where we explore the emergence of chaotic behaviour and revisit the role of the measurement process in the quantum-to-classical transition.
Demonstration of fidelity improvement using dynamical decoupling with superconducting qubits
Presenting Author: Bibek Pokharel, University of Southern California
Quantum computers must be able to function in the presence of decoherence. The simplest strategy for decoherence reduction is dynamical decoupling (DD), which requires no encoding overhead and works by converting quantum gates into decoupling pulses. Here, using the IBM and Rigetti platforms, we demonstrate that the DD method is suitable for implementation in today’s relatively noisy and small-scale cloud-based quantum computers. Using DD, we achieve substantial fidelity gains relative to unprotected, free evolution of individual superconducting transmon qubits. To a lesser degree, DD is also capable of protecting entangled two-qubit states. We show that dephasing and spontaneous emission errors are dominant in these systems, and that different DD sequences are capable of mitigating both effects. Unlike previous work demonstrating the use of quantum error correcting codes on the same platforms, we make no use of postselection and hence report unconditional fidelity improvements against natural decoherence.
Randomized benchmarking of many-qubit devices
Presenting Author: Timothy Proctor, Sandia National Laboratories
Quantum information processors incorporating 5 - 10s of qubits are now commonplace, but the standard method for benchmarking quantum gates - Clifford randomized benchmarking - is infeasible to implement on more than a few qubits in any near-term devices. In this talk, we present a series of modifications to Clifford randomized benchmarking that enable truly holistic benchmarking of entire devices. Importantly, these new techniques are adaptable based on experimental goals. They can be made highly robust or more scalable as needed, and they can be used to estimate, e.g., two-qubit gate error rates or the magnitude of crosstalk errors. Moreover, our methods allow for the benchmarking of universal gates, and continuously parameterized gates. We demonstrate our techniques on current systems, with experimental results on up to 16 qubits. Sandia National Labs is managed and operated by National Technology and Engineering Solutions of Sandia, LLC, a subsidiary of Honeywell International, Inc., for the U.S. Dept. of Energy’s National Nuclear Security Administration under contract DE-NA0003525. This research was funded by IARPA. The views expressed in the article do not necessarily represent the views of the DOE, IARPA, the ODNI, or the U.S. Government.
A computationally universal phase of quantum matter
Presenting Author: Robert Raussendorf, University of British Columbia
We provide the first example of a symmetry protected quantum phase that has universal computational power. Throughout this phase, which lives in spatial dimension two, the ground state is a universal resource for measurement based quantum computation.
High-efficiency demultiplexing of quantum information in temporal modes
Presenting Author: Michael G. Raymer, University of Oregon
Information can be encoded in single photons using temporal modes (sets of field-orthogonal wave-packet shapes). Temporal modes span a high-dimensional quantum state space and integrate into existing single-mode fiber communication networks, thus creating a new framework for quantum information science. A major challenge to achieving full control of temporal-mode states is their multiplexing and demultiplexing with zero crosstalk. Such add/drop functionality can be achieved by frequency conversion (FC) via nonlinear wave mixing, which can exchange the quantum states between two narrow spectral bands in a temporal-mode-selective manner. By tailoring the shape of the pump laser pulse and the phase-matching conditions of a second-order nonlinear optical medium, one can achieve moderate selectivity for different temporally orthogonal wave packets. To exceed this limit, we demonstrate a two-stage “Ramsey” interferometric FC scheme [1], predicted by theory to reach near-perfect (100%) selectivity. Using the two-stage scheme, we demonstrate a large increase over the single-stage selectivity limit, for the first three natural (“Schmidt”) modes of the FC process. This result paves the way for implementing arbitrary single-photon unitary operations, and thus various protocols such as QKD, in the temporal-mode basis. 1. “High-selectivity quantum pulse gating of photonic temporal modes using all-optical Ramsey interferometry,” D. V. Reddy and M. G. Raymer, Optica, 5, 423 (2018)
Characterizing errors in entangled-atom interferometry
Presenting Author: Brandon Ruzic, Sandia National Laboratories
Recent progress in generating entanglement between neutral atoms provides opportunities to advance quantum sensing technology. In particular, entanglement can enhance the performance of accelerometers and gravimeters based on light-pulse atom interferometry. We study the effects of error sources that may limit the sensitivity of such devices, including errors in the preparation of the initial entangled state, spread of the initial atomic wave packet, and imperfections in the laser pulses. Based on the performed analysis, entanglement-enhanced atom interferometry appears to be feasible with existing experimental capabilities.
Quantum algorithms for systems of linear equations inspired by adiabatic quantum computing
Presenting Author: Yigit Subasi, Los Alamos National Laboratory
We present two quantum algorithms based on evolution randomization, a simple variant of adiabatic quantum computing, to prepare a quantum state \(ket{x}\) that is proportional to the solution of the system of linear equations \(A \vec{x}=\vec{b}\). The time complexities of our algorithms are \(O(\kappa^2 \log(\kappa)/\epsilon)\) and \(O(\kappa \log(\kappa)/\epsilon)\) where \(\kappa \) is the condition number of \(A\) and \(\epsilon\) is the precision. Both algorithms are constructed using families of Hamiltonians that are linear combinations of products of \(A\), the projector onto the initial state \(|b>\), and single-qubit Pauli operators. The algorithms are conceptually simple and easy to implement. They are not obtained from equivalences between the gate model and adiabatic quantum computing, and do not use phase estimation or variable-time amplitude amplification.
We describe a gate-based implementation via Hamiltonian simulation and prove that our second algorithm is almost optimal in terms of \(\kappa \).
Like previous methods, our techniques yield an exponential quantum speed-up under some assumptions. Our results emphasize the role of Hamiltonian-based models of quantum computing for the discovery of important algorithms.
Exact entanglement cost of quantum states and channels under PPT-preserving operations
Presenting Author: Mark Wilde, Louisiana State University
This paper establishes single-letter formulas for the exact entanglement cost of generating bipartite quantum states and simulating quantum channels under free quantum operations that completely preserve positivity of the partial transpose (PPT). First, we establish that the exact entanglement cost of any bipartite quantum state under PPT-preserving operations is given by a single-letter formula, here called the κ-entanglement of a quantum state. This formula is calculable by a semidefinite program, thus allowing for an efficiently computable solution for general quantum states. Notably, this is the first time that an entanglement measure for general bipartite states has been proven not only to possess a direct operational meaning but also to be efficiently computable, thus solving a question that has remained open since the inception of entanglement theory over two decades ago. Next, we introduce and solve the exact entanglement cost for simulating quantum channels in both the parallel and sequential settings, along with the assistance of free PPT-preserving operations. The entanglement cost in both cases is given by the same single-letter formula and is equal to the largest κ-entanglement that can be shared by the sender and receiver of the channel. It is also efficiently computable by a semidefinite program.
Towards spin-squeezed matter-wave interferometry
Presenting Author: Baochen Wu, University of Colorado JILA
Quantum entanglement permits the creation of spin-squeezed states where the fundamental quantum noise of one atom can be partially cancelled by another atom. Spin-squeezed states are particularly promising for enhancing precision measurements beyond the standard quantum limit for unentangled atoms. We have previously demonstrated 18 dB of squeezing (Cox et al, PRL, 116, 093602) with cavity-assisted non-demolition measurements in 87Rb atoms. Here we present our recent efforts towards building an intracavity, guided matter-wave interferometer in which spin-squeezing will be mapped to momentum states. This could help pave the way for better determinations of fundamental constants, more precise inertial sensors, and enhanced searches for dark matter.
General modeling framework for quantum photodetectors
Presenting Author: Steve Young, Sandia National Laboratories
Photodetection plays a key role in basic science and technology, with exquisite performance having been achieved down to the single photon level. Further improvements in photodetectors would open new possibilities across a broad range of scientific disciplines, and enable new types of applications. However, it is still unclear what is possible in terms of ultimate performance, and what properties are needed for a photodetector to achieve such performance. Here we present a general modeling framework for single- and few- photon detectors wherein the entire detection process - including the photon field, environmental coupling, and measurement output - is treated holistically and quantum mechanically. The formalism naturally handles field states with single or multiple photons as well as arbitrary detector configurations. It is explicitly constructed to provide performance characteristics and naturally furnishes a mathematical definition of ideal photodetector performance. The framework reveals how specific photodetector architectures and physical realizations introduce limitations and tradeoffs for various performance metrics, providing guidance for optimization and design.